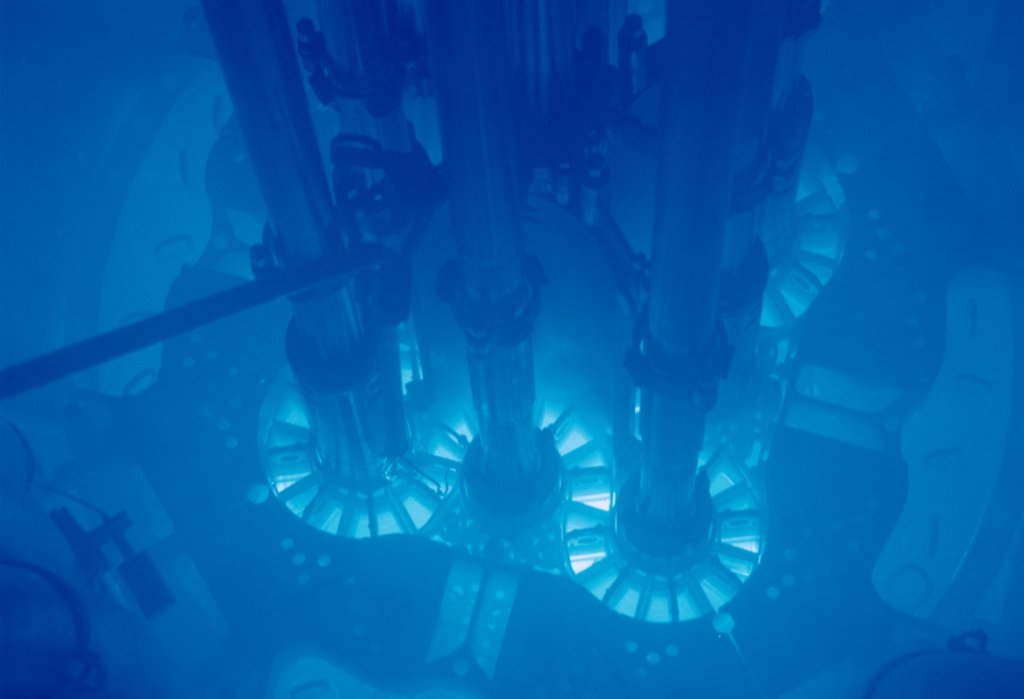
Cherenkov radiation glowing in the core of the Advanced Test Reactor at Idaho National Laboratory. By Argonne National Laboratory – originally posted to Flickr as Advanced Test Reactor core, Idaho National LaboratoryUploaded using F2ComButton, CC BY-SA 2.0, https://commons.wikimedia.org/w/index.php?curid=27024528
Nuclear model of the atom
What is radiation?
The word radiation arises from the phenomenon of waves radiating (i.e., traveling outward in all directions) from a source.
In physics, radiation is the emission or transmission of energy in the form of waves or particles through space or through a material medium.
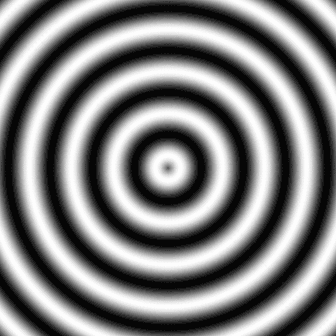
The image above shows radiating waves.
The image below shows thermal or heat radiation.
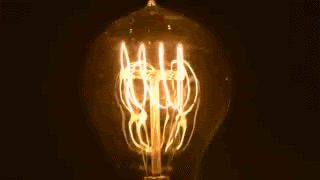
Radiation carries energy.
What is radioactivity?
The emission of radiation (particles and energy) from atoms is called radioactivity.
Radioactivity can be natural.
The image shows trails caused by radiating particles from a lump of uranium.
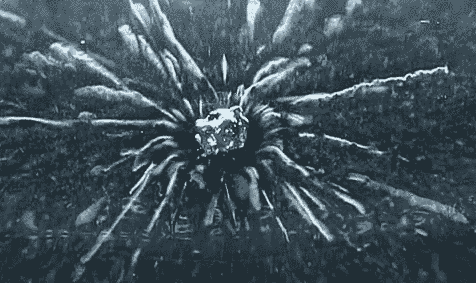
What is nuclear radiation?
Nuclear radiation is simply radiation that is emitted from the nucleus of an atom.
Substances that release nuclear radiation are described as being radioactive. They are called radioisotopes.
To understand radioisotopes, we need to learn about isotopes.
To understand isotopes, we need to understand the structure of the nucleus
Atomic structure and isotopes
What are isotopes?
Isotopes are two or more types of atoms that have the same number of protons but have different numbers of neutrons in their nuclei.

By Dirk Hünniger;Derivative work in english – Balajijagadesh – This file was derived from: Hydrogen Deuterium Tritium Nuclei Schmatic-de.svg, CC BY-SA 3.0, https://commons.wikimedia.org/w/index.php?curid=46295940
Unstable isotopes are radioactive and called radioisotopes.
What is relative atomic mass?
The numbers on the Periodic Table that you’re given in exams do not include mass numbers! They are standard relative atomic masses (also known as atomic weights). You can round to find the most common mass number.
Watch the video below to find out how mass number and relative atomic masses are different. Note that the video refers to Atomic Weight instead of Relative Atomic Mass but the terms can be used interchangeably.
The relative atomic mass of an element is the ratio of the weighted average mass per atom of the naturally occurring form of the element to 1/12 the mass of an atom of carbon-12 (TASC 2022)
The relative atomic mass of an element is given by:
where m1, m2, …, mN are the relative atomic masses of each individual isotope, and x1, …, xN are the relative abundances of these isotopes.
Why is it a relative atomic mass? The mass value is considered relative to that of carbon-12. For this reason it has no units.
What is a mass spectrometer?
Mass spectrometers are used to determine which atoms or molecules are in a sample of material. Mass spectrometers are used in airport security and forensic analysis.
You need to be able to answer the following questions:
- What are the steps involved in mass spectrometry?
- What is the result after analysis using mass spectrometry?
- How do mass spectrometers provide evidence for the existence of isotopes?
How does a mass spectrometer work?
- mass spectroscopy involves the ionisation of substances and their separation using a magnetic field (TASC)
The main steps are:
- Vaporisation of the sample
- Ionisation of the sample
- Deflection using a magnetic field
- Detection
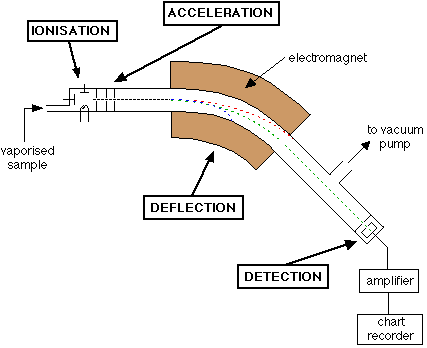
An example mass spectrogram
The diagrams produced are called mass spectrograms, mass spectrographs or simply mass spectrums!

What is the horizontal axis of a mass spectrograph?
A mass spectrum will usually be presented as a vertical bar graph, in which each bar represents an ion having a specific mass-to-charge ratio (m/z) and the length of the bar indicates the relative abundance of the ion.
The horizontal axis is either mass-to-charge ratio or relative isotopic mass. (Because most of the ions formed in a mass spectrometer have a single charge, so the m/z value is equivalent to mass itself.)
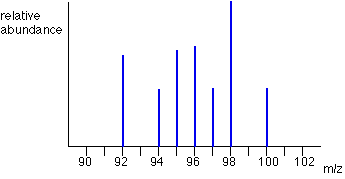
What is the vertical axis of a mass spectrograph?
Sometimes, relative abundance is measured as a percentage. The mass spectrum for zirconium is shown. These graphs are easy to interpret.
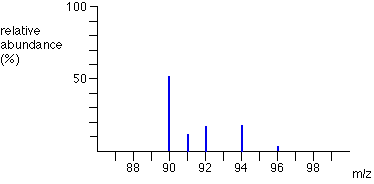
Sometimes, the vertical axis is labelled as either “relative abundance” or “relative intensity” but it’s not a percentage. The most intense ion is assigned an abundance of 100, and it is referred to as the base peak. E.g. the mass spectrum of boron is shown. How to interpret these graphs is shown on the next slide.
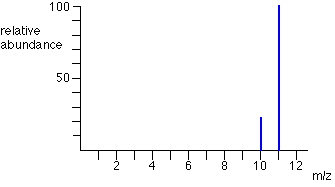
How to interpret mass spectrographs?
The two peaks in the mass spectrum for a sample of boron shows that there are 2 isotopes.
The two isotopes and their relative abundances are :
- Boron-10: 23
- Boron-11: 100
To calculate the relative abundance as a percentage, divide each relative abundance by the total abundance:
- Boron-10: 23/123 = 18.7 %
- Boron-11: 100/123 = 81.3 %
Calculate Boron’s relative atomic mass, Ar.
What does the mass spectrum of a molecule look like?
Previous mass spectra have been for elements (and not diatomic molecules)
When molecules are passed into the ionisation chamber, an electron is knocked off the molecule to give a molecular ion, e.g. CO2+. These ions won’t be particularly stable, and some will fall apart to give fragments. In this example: ions of O and CO are produced.
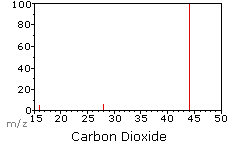
The oxygen ion is the peak at 16 and the CO ion is the peak at 28. The molecular CO ion is the peak at 44.
How do mass spectrometers provide support for the existence of isotopes?
If a sample contains isotopes of an element, the mass spectrometer will produce, separates and detect ions of the element of slightly different masses.
The presence of different types of an element with different masses can be explained if the element exists in versions with different numbers of neutrons (isotopes).
Types of nuclear radiation
What is radioactive transmutation?
In 1902, Rutherford and Soddy proposed that the emission of radioactivity was the result of ‘radioactive transmutation’. When a radioactive atom emits an alpha (⍺) particle or beta (𝛽) particle, the atom split into two. After the ⍺ particle or 𝛽 particle was emitted, what remained was a heavy leftover part that was chemically and physically different from the parent atom.
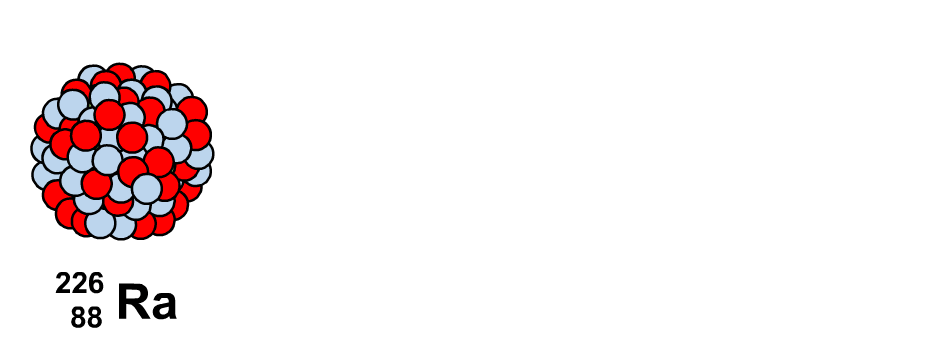
This ‘transformation’, ‘disintegration’, ‘decay’ or ‘transmutation’ was responsible for turning one element into another.
What is nuclear radiation?
Later, it was realised that the radiation emitted during transmutation is emitted from the nucleus, so it was referred to as nuclear radiation.
Why are some isotopes radioactive?
Atoms emit radiation because they are unstable.
Unstable nuclei randomly and spontaneously emit particles or waves that carry energy away from the nucleus.
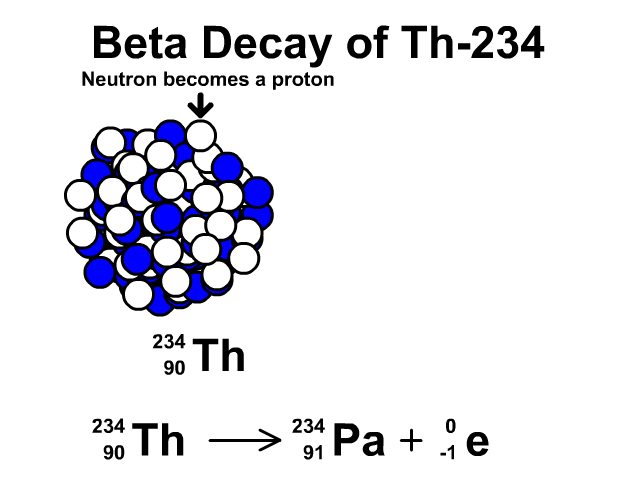
What is the difference between ⍺, 𝛽 and 𝛾 radiation?
In the years 1899 and 1900, Rutherford and Villard separated radiation into three types: eventually named alpha, beta, and gamma by Rutherford, based on their ability to penetrate of objects and their deflection by a magnetic field.
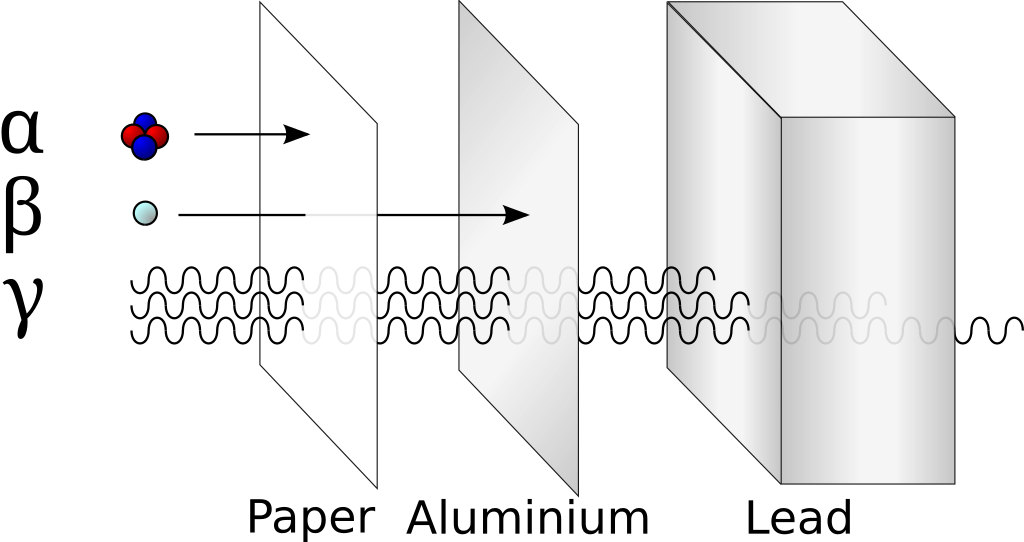
Ionisation is the formation of ions (charged atoms). The more penetrating the radiation, the less ionising.
The ionising power is the inverse of the penetrating power.
Alpha particles interact most strongly with matter and hence have a low penetrating power and high ionising power. The ionising power of beta particles is lower and that of gamma rays is very low.
The ionising power of gamma rays depends on the energy of the photons.
How do alpha particles cause ionisation?
Alpha particles ionise atoms by directly interacting with their outer electrons via the electrostatic force. Since the particle is positively charged, it exerts an attractive force on the oppositely charged electron. The attractive force is sufficient to remove the electron from the atom (ionization).
The closer an alpha particle passes near an electron the stronger the force and the higher the probability an ionizing event will occur. In these situations, the electron may be imagined as being “ripped” from its orbit as the alpha particle passes nearby.
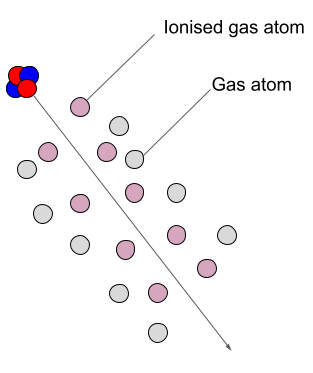
How does radiation cause ionisation?
In air, alpha particles can produce about 10,000 ion pairs per centimeter or more.
Alpha particles lose energy with each interaction until finally picking up two electrons to form a neutrally charged Helium atom.
Beta particles also ionise atoms by directly interacting with them via the electrostatic force. A beta particle may collide with an outer shell electron or come into close proximity to it and cause the electron to be ejected, thereby resulting in the formation of an ion pair.
Gamma rays can cause ionisation via the photoelectric effect or other interactions not in our course (e.g. the Compton effect)
Nuclear reactions
How do we describe a nucleus?
The number of protons in a nucleus is denoted by Z, and is called the atomic (or proton) number.
The total number of nucleons (protons + neutrons) is called the mass (or nucleon) number, and is denoted by A.
The number of neutrons in a nucleus is denoted by N with N = A − Z.
The term nuclide means a nucleus characterised by a particular atomic number and mass number (Z and A numbers).
This term is used on the assignment sheets and might be used in exam questions.
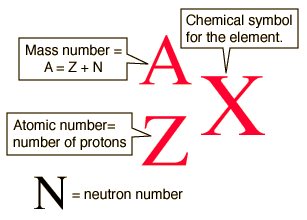
How can we represent the subatomic particles?
We can apply this notation to the nucleons and electrons. For example, the proton (symbol p) can be written as:
The neutron (symbol n) can be written as:
The mass number of the electron is zero – as it is neither a proton nor a neutron, and the mass number is defined as the total number of neutrons plus protons. If we notice that the atomic number is not only the number of protons in the nucleus but also its electric charge in units of e, then we can extend this notation to electrons as well. The charge of the electron in units of e is −1 and so we represent the electron by:
How can we represent other particles?
Sometimes, particles other than protons, neutrons and electrons are represented in a similar way, especially when they participate in nuclear reactions.
For example, the photon (the particle of light) has the Greek letter gamma as its symbol. Since it has zero electric charge and is neither a proton nor a neutron, it is represented by:
All of these symbols are in your information booklet.
How do we write the formulae for nuclear reactions?
Nuclear reactions obey conservation laws:
- The law of conservation of mass/energy
- The law of conservation of charge.
- The number of nucleons is also always conserved.
Thus, in any nuclear reaction:
- the sum of the mass numbers before the reaction must be equal to the sum of the mass numbers after the reaction (this shows conservation of nucleons)
- The sum of the atomic numbers before the reaction must likewise be equal to the sum of the atomic numbers after the reaction (this shows conservation of charge).
If a beta decay is involved, the beta particle is assigned a positive or negative atomic number to account for conservation of charge. This is simply an accounting method – electrons don’t really have a negative atomic number.
How to describe alpha decay?
After the nucleus has emitted an alpha particle the element changes and:
- the mass number A decreases by four, corresponding to the loss of two protons and two neutrons,
- the atomic number Z decreases by two, corresponding to the loss of two protons,
- the number of neutrons N decreases by two, corresponding to the loss of two neutrons.
The general equation for this process is:
Where X is the parent and Y is the daughter isotope
For example, a common naturally occurring alpha decay is that of Uranium-238 decaying to Thorium-234:
How to describe beta-minus decay?
During β– decay, a neutron transforms into a proton and an electron (a β– particle). After β– decay, the element changes and:
- The mass number A remains the same, as the total number of protons and neutrons is unchanged.
- The atomic number Z increases by one
- The number of neutrons N decreases by one, corresponding to the transformation of a neutron into a proton.
Thus, the generic equation for this process is:
For example, the decay of Thorium-234 to Protactinium-234:
How to describe gamma decay?
In gamma decay a nucleus emits a gamma ray, in other words a photon of high-frequency electromagnetic radiation. After gamma decay, the element does not change and:
- The mass number A remains the same, as the total number of protons and neutrons is unchanged.
- The atomic number Z remains the same
- The number of neutrons N remains the same
- The nucleus becomes more stable by moves from a higher to a lower nuclear energy level.
The generic equation for this process is:
For example, Uranium-238 undergoes gamma decay:
Typical half-lives for gamma emission are immeasurably short (from about 10-9 to 10−14 second). (Britannica 2020)
What are metastable states?
When the nucleus is in an excited state it can lose energy and become more stable by releasing gamma rays.
Metastable isotopes decay via gamma decay.
When the nucleus is in a metastable state, it has a longer half life than other unstable nuclei which emit gamma rays ‘promptly’.
We indicate metastable nuclei using “m” notation:
When is gamma radiation released?
A radioactive nucleus can decay by the emission of an alpha or beta particle. The daughter nucleus that results is usually left in an excited state (an unstable, higher energy state). It can then decay to a lower energy state by emitting a gamma ray photon (gamma decay)
Gamma decay normally occurs after other forms of decay occur, such as alpha or beta decay.
Excited nuclear states are usually very short lived, decaying almost immediately after alpha or beta decay.
Thus, Gamma emitters (like Co-60; see diagram) usually emit gamma rays at virtually the same time as alpha or beta particles.
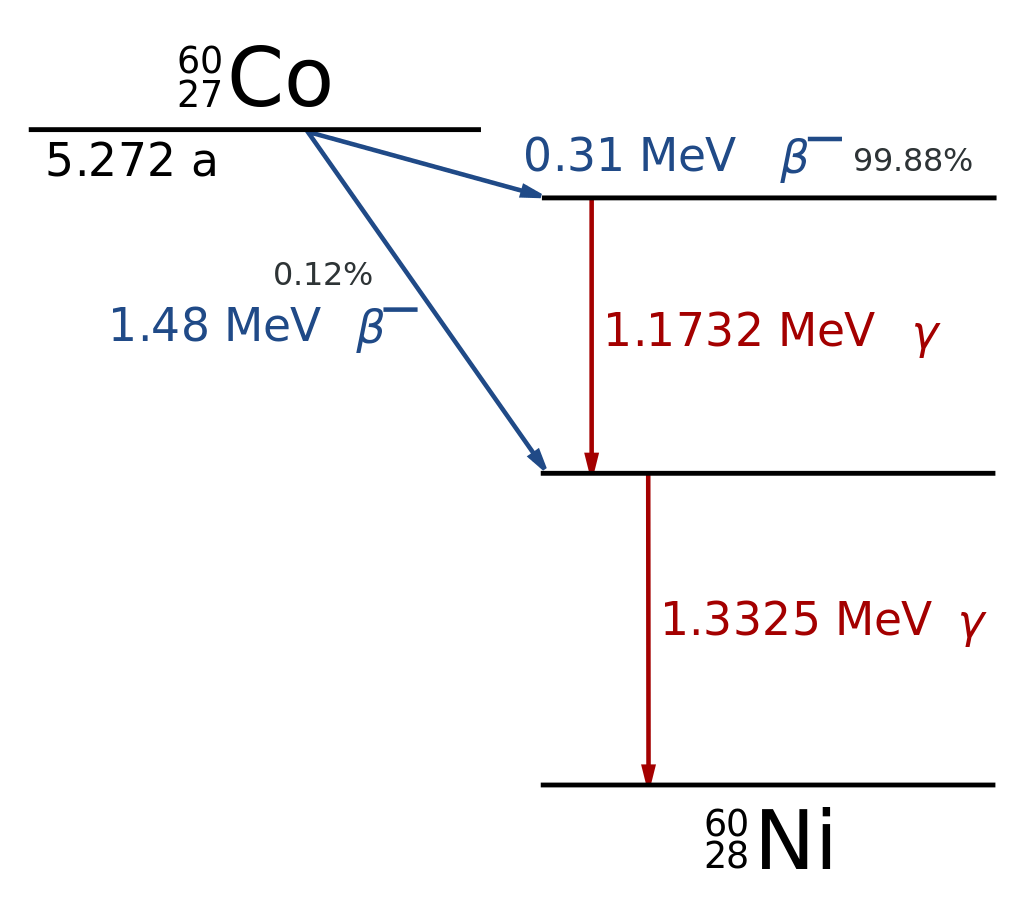
By Inductiveload – This W3C-unspecified vector image was created with Inkscape ., Public Domain, https://commons.wikimedia.org/w/index.php?curid=2859326
What are the advantages of metastable isotopes?
Metastable isotopes are “pure” gamma emitters (as a consequence of their relatively long half-lives).
Unlike Co-60, which is used as a gamma source, Tc-99m only emits gamma rays.
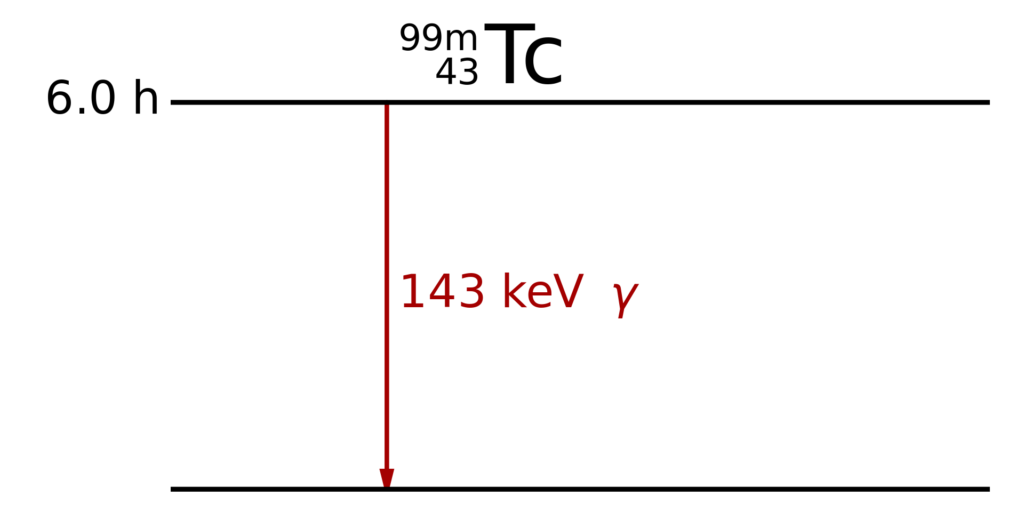
The metastable isotope of Technetium decays after a half-life of 6.0 hours by a single 143 keV gamma emission. By Inductiveload – This W3C-unspecified vector image was created with Inkscape ., Public Domain, https://commons.wikimedia.org/w/index.php?curid=9781258
Pure gamma emission is the desirable decay mode for medical imaging because other particles deposit more energy in the patient body (radiation dose) than in the camera. Metastable isomeric transition is the only nuclear decay mode that approaches pure gamma emission. (Wikipedia)
Artificial nuclear reactions, Nuclear fission and chain reactions
How to produce nuclear reactions?
The radioactivity that we have encountered so far has been associated with natural alpha, beta and gamma emitters. Rutherford was the first to use alpha particles to produce nuclear reactions.
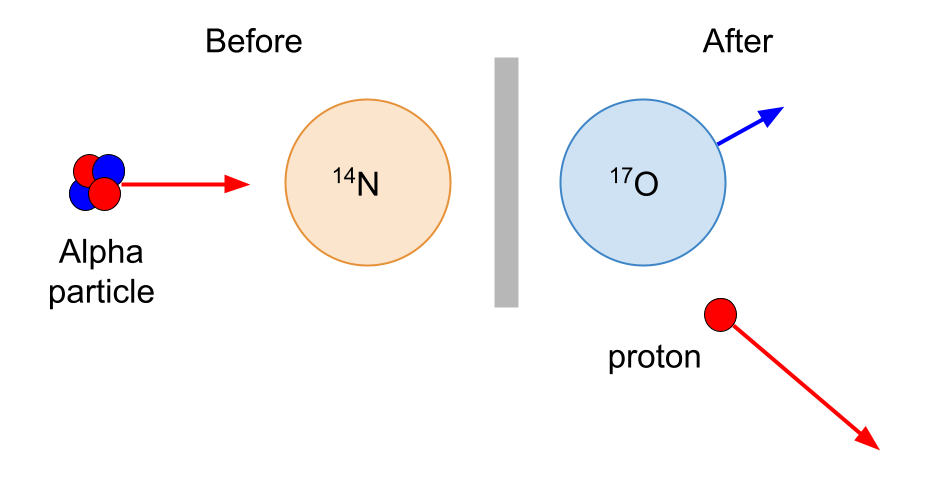
In 1919, Rutherford bombarded nitrogen gas with alpha particles from bismuth-214. A positively charged particle which was more penetrative than an alpha particle was produced. This particle was identified as a proton. The nitrogen is transformed into oxygen.
Note that this is a nuclear reaction but it is not a type of nuclear decay. It is induced, not spontaneous.
The alpha particle had combined with the nitrogen nucleus and a proton had been emitted. The alpha particles from the bismuth-214 source were able to approach the nucleus very closely and occasionally make contact with it.
What is nuclear fission?
Nuclear fission is the splitting of a large atomic nucleus (Z > 56) into smaller fragments. It usually releases neutrons and large amounts of energy.
Nuclear fusion is triggered by the absorption of a ‘slow’ (5 – 10 keV) neutron. It involves the release of large amounts of energy.
This energy can be used to drive turbines and produce electricity in nuclear power plants.
How is nuclear fission different to radioactive decay?
What is a chain reaction?
If the fission fragments include two or more neutrons, these neutrons can cause other nuclei to split, resulting in a self-sustaining chain reaction.
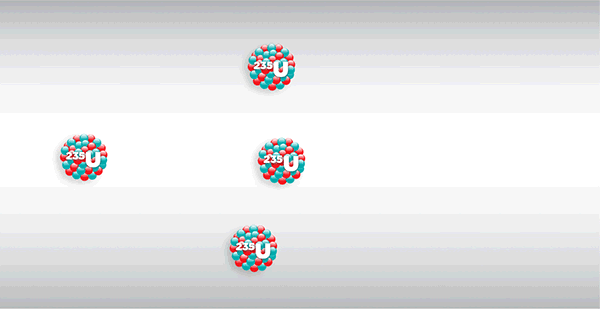
https://mag.uchicago.edu/science-medicine/manhattans-critical-moment
What’s the difference between controlled and uncontrolled reactions?
A nuclear chain reaction can rapidly multiply to huge numbers of fission events. Unless this is carefully controlled, a runaway explosion will occur.
In a controlled chain reaction, one fission event releases 2 or 3 neutrons, but only one must be allowed to cause fission in another uranium nucleus.
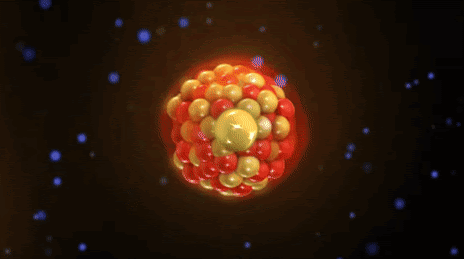
If the neutrons released cause more than one fission event on average, then a runaway reaction occurs. If the average number of fission events caused by the neutrons released is less than one, the reaction dies out.
How are controlled and uncontrolled reactions used?
The heat generated by fission reactions can be used by humans.
If the fission reactions are controlled, then we can harness the heat in nuclear power stations and use it to make electricity.
If the fission reactions are uncontrolled, then the heat can be used to make weapons of mass destruction.
Half-life and decay rate
What are the characteristics of radioactive decay?
Radioactive decay is random and spontaneous.
By random we mean that we cannot predict which unstable nucleus in a sample will decay or when there will be a decay. It is spontaneous because we cannot affect the rate of decay of a given sample in any way.
Although we cannot predict or influence when a particular nucleus will decay, we know that the number of nuclei that will decay per second is proportional to the number of nuclei in the sample that have not yet decayed.
What is the pattern of radioactive decay?
A consequence of this law is that the number of radioactive nuclei decreases exponentially.
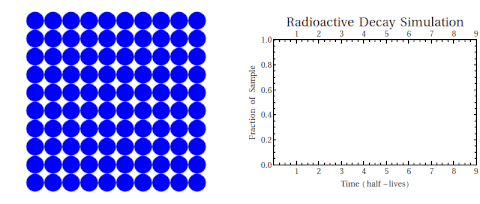
Radioactive thallium decays into lead:
The graph shows how the number of thallium nuclei decreases with time. Initially (at t = 0) there are 1.6 × 1022 nuclei of thallium. After 3 min the number of thallium nuclei left is half of the initial number. After another 3 min, the number is one-quarter of the initial number. After yet another 3 min the number of thallium nuclei is one-eighth of the initial number.
What is the half-life of an isotope?
The time of 3.0 min is called the half-life of thallium. It is the time after which the number of the radioactive nuclei is reduced by a factor of 2.
Simply put: the half-life of a radioisotope is the time required for half of the atoms in the original sample to decay.
Every radioisotope has a unique half-life.
As the parent isotope in a sample decreases, the daughter isotope accumulates.
How to calculate the amount of parent isotope remaining?
If the number of half lives, n, that have elapsed is known, then we can use the concept of half-life to calculate the amount of parent isotope remaining at that time, Nt:
This formula states that after one half-life, (½)1 of the original sample remains.
After two half-lives, (½)2 = (½)×(½) = ¼ of the original sample remains
After three half-lives, (½)3 = (½)×(½)×(½) = ⅛ of the original sample remains
What is the activity of sample?
A concept that is useful in experimental work is that of decay rate or activity A: this is the number of decays per second.
We cannot easily measure how many unstable nuclei are present in a sample, but we can detect the decays. The unit of activity is the becquerel (Bq): 1 Bq is equal to one decay per second.
Activity obeys the same exponential decay law as the number of nuclei: In a time equal to the half-life the activity is reduced by a factor of 2.
Thus, we can use activity to determine the half-life of a radioisotope.
Why is background radiation important?
In the graph on the previous slide the activity approaches zero as the time increases. In practice, however, this is not the case.
The detector that measures activity from the radioactive sample under study also measures the activity from natural sources, including cosmic rays from the Sun, radioactive material in rocks and the ground, radiation from nuclear weapons testing grounds, and so on.
As a result, the activity does not approach zero; it approaches the activity due to all other sources of background radiation.
What is the background radiation measured by the detector which produced the graph shown? What is the half life of the radioisotope?
References
J.W. Poston Sr., in Encyclopedia of Physical Science and Technology (Third Edition), 2003.
https://www.sciencedirect.com/topics/physics-and-astronomy/alpha-radiation#